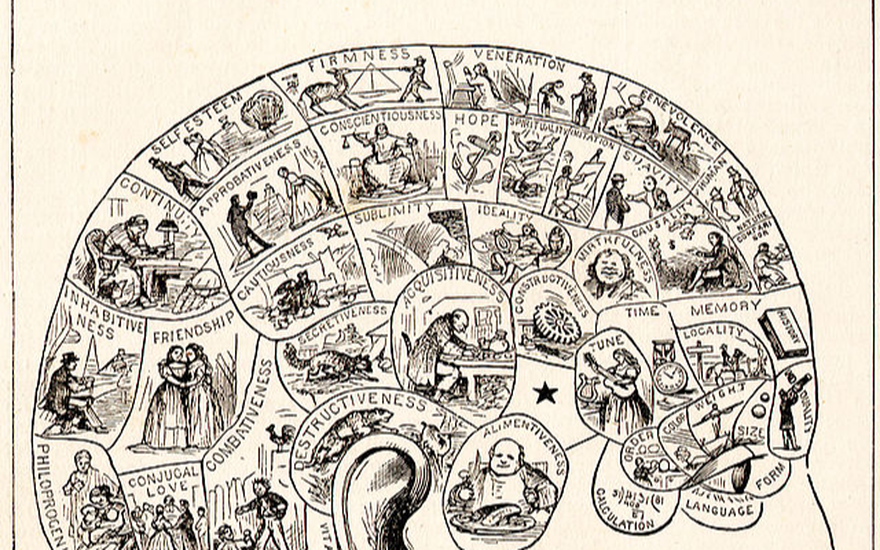
Public Domain image from Wikimedia Commons
Science of Chess - Finding chess expertise in the brain: A case study
What do we learn by looking for neural networks that support chess expertise in the human brain? What do we find when we do?So far in these "Science of Chess" articles, I've focused on various research results describing the cognitive and perceptual science of chess. You could broadly summarize all of these essays as attempts to comment on what we know about how chess works in the mind. With the fall semester at my home institution rapidly approaching (where did the summer go?), however, I'm working on the final touches for the upper-division course I offer in Neuropsychology. This means thinking not just about the mind, but also about the brain. I love teaching this course, but it also gives rise to a lot of mixed feelings for me. I want to spend a little bit of time here at the outset of this article explaining why, which I hope will be a useful preamble to the study (by Cepero-Escribano and colleagues) that I want to tell you about.
Who cares about the brain anyway?
The thing is, I'm sort of a brain skeptic. Oh sure, I know that all the cognitive functions I'm interested in are supported in one way or another by the nervous system - that part's fine. The thing that I tend towards the skeptical about is the value added by using the tools of cognitive neuroscience to try and understand the nature of those processes. I like to think that I come by this skepticism honestly: I was raised (academically speaking) in a very active fMRI lab and did post-doctoral work with EEG/ERP as part of a different (and also very active) research group. I know what these methodologies allow you to do and I've used them myself to try and understand things like the effects of visual experience of recognition abilities or what critical features contribute to specific kinds of visual processing developmentally. I think these techniques absolutely have their place and can help us understand fundamental questions about what the mind and the brain are doing in different settings. If you look around my lab these days, though, you'll see a lot more emphasis on analog tools for running experiments: My undergrads have been running coloring and drawing tasks, asking kids and adults to put stickers on shape silhouettes, and a bunch of other stuff that has little connection to the world of Cog. Neuro. Ultimately, though I sort of built my lab up via electrophysiology, I found myself getting increasingly worried about relying too heavily on these methods.
The thing that I started to worry about, with regard to my academic discipline but especially with regard to my students, is that it's easy to get sort of taken in by what some have called the "seductive allure" of brain imaging. I remember the first time I got the chance to run a simple analysis on real fMRI data and ended up with bright blobs of color superimposed on an image of a real participant's brain. It felt a little like magic and it looked the way that I thought science was supposed to look. I'd end up joining the instructor's lab as an undergraduate and would eventually take part in enough fMRI studies to have a pretty specific idea about where my own face processing network is inside my skull and an image of my brain that I still use when I teach (see below). Cool, right?
Figure 1 - A mid-sagittal view of NDpatzer's brain (circa 2001 or so).
Yes - it is cool. But like all methods and all experimental designs, it requires careful thought at all stages. It's not enough to just treat the brain as an expensive dependent variable! For me, the thing I try to emphasize when I teach my Neuropsych course is one question above all: What do we get out of studying the brain directly that we couldn't get by other means? There are a lot of reasons that this question is important to ask, including one's budget (which is probably smaller than you want), participants' time (which they would like to mostly spend outside of an fMRI scanner), and other practical considerations. Look, why use all this money and time and energy to go poking around the cortex? Who cares about the brain, anyway?
My students generally don't like this question because they think the idea of being a neuroscientist is neat. But why is it neat? Some of my students reply to this by saying things like "Then you know where stuff happens in the brain." to which I usually reply "So?" That always bring them up a little short because it sure seems like something worth finding out. But is it? If I told you chess expertise depended critically on a 4mm x 4mm chunk of cortex in the right frontal lobe, would this change anything for you? I mean, you could use this to start making the sort of phrenological mapthat 18th and 19th century scientists were fond of. Once you had such a chart, though, what is it for? Do you know more than just a bit of geography to attach to the various things your mind can do?
My students very much feel like the answer to that question has to be "yes," but we spend a lot of time examining why they feel this way. I still think a lot about this paper from Weisberg et al. in 2008 in which they presented their participants with Good and Bad explanations for various psychological outcomes, some of which included no neuroscience content at all and others that did. Critically, the neuroscience that was included was totally irrelevant. Even so, what they found was that just having some neuroscience hanging around didn't have any effect on participants' perceptions of good explanations, but it made bad ones seem better.
Figure 2 (adapted from Weisberg et al., 2008) - Dropping some irrelevant neuroscience into the explanation of a psychological phenomenon makes a poor explanation seem better.
This is what I feel like I'm obligated to fight against when I teach Neuropsychology. I usually start the semester by buying a few different products from our campus convenience store that advertise "Brain-boosting" somethingsomething to try and make this point a little more forcefully - it's easy to be fooled into thinking that "neuro-" anything must be important and deep. Getting the brain involved isn't inherently meaningful, however, and knowing where something appears to happen in the brain might not really inform your understanding of the phenomenon you care about.
The thing is, of course, that it also might do so - but that depends on how you've designed your study and how you're planning to use the brain to draw conclusions. Let me give you just two reasons you might give for being interested in that little (fake!) factoid I just gave you: (1) You're interested in the brain itself as your object of study, and this is a new bit of data to add to the catalog of stuff you know about this organ. (2) You already know some things about how the brain is organized into functions, so finding out that chess expertise "lives" here helps you link it to other cognitive processes. In response to (1) I say, "Sure - good on you. Not my cup of tea, but not everything needs to be." To (2), I invite you out for coffee because we have a lot to talk about. See, at least for me, that's the thing that's interesting about examining the link between mind and brain - understanding how processes are organized cortically can often give you insights about how the true nature of different behaviors and abilities. What is chess? What is it that we're actually doing when we look at a board and try to win a game or solve a puzzle? You can introspect all you want about what you feel like you're doing, but neural data provides a means of carving the space of human behaviors, perceptions, and thoughts at a set of joints defined by the way our biological hardware is applied to different tasks.
This isn't the only or best perspective to have on cognitive neuroscience, but it's mine. What I'm hoping I can do by taking a look at the cognitive neuroscience of chess is show you how data from the human brain can help complement our understanding of how the mind plays the game. Here, I'd like to do that by looking at a study that I think is interesting to consider because it offers a third answer to my question about why you might want to look for a particular function in the brain: What if you needed to do so to try and protect it?
Searching for eloquent cortex with fMRI
Allow me to introduce you to the subject of our case study, Patient AB. Part of the reason this study stood out to me was that I couldn't help but think AB sounded a lot like someone I could have met in college: 45 years old, a skilled programmer, multi-lingual, and a chess player with a pretty solid rating of 1950 ELO. Besides these traits and abilities, AB also had something else: A headache. Not just any headache, either, but a headache caused by a type of brain tumor called a Glioblastoma. You can see what his tumor looked like in the top row of the figure below - I'd say something to get you oriented towards what to look for, but I have a hunch you'll work it out on your own. While we're here, I'll also get straight to the point and refer you to the bottom row of the figure, too: The thing that AB's surgeons had to do was turn the brain that you see in the top row into the brain that you see in the bottom row. That is, the tumor would have to be resected.
Figure 3 (adapted from Cepero-Escribano et al., 2024) The top row depicts Patient AB's brain pre-operatively, with a large glioblastoma evident in the LH. The bottom row depicts the results of the surgery to remove this region. How do you make sure that the transition from the top row to the bottom row doesn't negatively impact AB's cognition?
This is no small thing to do, though. The obvious concern is that removing the tumor may also mean removing eloquent cortex, or portions of the brain that support specific functions like movement, sensation, or cognition. Remember all those things that I said AB was able to do? All of these were things that he also hoped to be able to do after the surgery - including playing chess at a fairly high level (much higher than your humble narrator at least). So what to do? I bring this kind of scenario up to my students a lot to remind them both that neuropsychology as a discipline is built on things we have learned from real people facing difficult circumstances imposed by injury or disease, and also that assessing cognitive abilities is difficult and important. How do you measure any complex ability like chess-playing or programming skill in a meaningful way? We have existing neuropsychological scales to assess cognition broadly in terms of memory, visual perception, and language, but what do you do to help a particular person preserve their specific skills when they have to undergo surgery on their brain? What the authors of this case study did for AB is quite cool, helping to ensure the preservation of his cognitive abilities while also offering some additional insights into what chess playing is in terms of a definition based on neural systems.
The big idea is that this is absolutely a case in which it is imperative to know where different kinds of processes are carried out by AB's with as much geographical specificity as you can come up with. That means linking structure to function closely enough that you understand how to plan surgical incisions around portions of his neural network that implement behaviors you want to leave intact. But how? One obvious candidate is to use fMRI (functional magnetic resonance imaging), which provides an indirect measure of neural activity in the brain via the measurement of blood oxygenation Briefly, active neurons require oxygen, which is released by oxygenated hemoglobin. This means that brain areas with high activity will end up with measurable differences in the amounts of oxygenated- vs. deoxy-hemoglobin and an fMRI scanner measures this via something called the BOLD, or Blood Oxygenation Level Dependent, response. When you see those pretty "heat-maps" of fMRI data, that's what you're being shown, but with an important caveat: fMRI analyses are generally based on statistical comparisons between the BOLD response observed under different conditions. That is, we are typically not just seeing where the brain "lights up" when you do some task, but are instead seeing portions of the brain where there is a significant difference between the BOLD response in one condition vs. another condition. This matters because you have to keep in mind that nearly anything you do doesn't rely on just one brain area, but recruits many different parts of your brain. We can see how this works by looking at some data from Patient AB based on different contrasts, or comparisons between tasks administered while he was in an fMRI scanner during pre-operative testing. First, let's take a look at the different chess tasks that they asked him to do. Below, I'm showing you a set of 5 different behavioral tasks they asked him to carry out that spanned a range of different cognitive abilities involving chess pieces. Some of these only required knowing what the pieces were called - Tasks 1 and 2 are just tests that involve finding and naming pieces on the board. Others required more of what we might call actual chess knowledge - Task 3 is a judgment about legal moves, for example, while Task 4 is an assessment of Mate-in-2 potential.
Figure 4 (adapted from Cepero-Escribano et al., 2024) - Examples of the behavioral tasks administered to Patient AB during pre-op behavioral testing (so not in the scanner).
But now let's take a look at the things they asked him to do while the measured the BOLD response in the scanner. These should look familiar because Conditions B,C, and D are just scanner-friendly versions of some of the behavioral tasks I showed you in the previous figure. There is something new here, though, tucked away in the bottom-left corner of the figure: A little do-nothing condition called "Resting" which is exactly what it sounds like - Patient AB hangs out for a bit not doing anything in particular. This simple design means that we get to see a few different answers to the question "Where is chess playing ability in AB's brain?" via different BOLD response contrasts. Let's start with a very broad one: What parts of the brain are more active when you're playing chess than when you're doing...well, nothing?
Figure 5 (adapted from Cepero-Escribano et al., 2024) - The tasks administered to Patient AB in the scanner. Note the new "Resting" condition, which serves as a sort of baseline for not doing anything in particular.
The image below shows you the network of regions you get when you look for parts of the brain that were significantly more active during any/all of the chess tasks (Conditions B-D all summed together) compared to the Resting condition. If it looks complicated - it is! Remember that this represents all the different things that are happening when AB carries out those chess tasks and that aren't happening when he's just staring a small plus sign for about 12 seconds. He's seeing colors and shapes (hello there, visual cortex!), he's making decisions about what to respond (looking at you frontal lobe and maybe some pre-motor cortex) and he's doing whatever spatial reasoning he needs to do to assess checkmating patterns and move legality. Where is chess in AB's brain? Kind of all over the place!
Figure 6 (adapted from Cepero-Escribano et al., 2024) - A contrast between all of the chess tasks administered in the scanner to AB and a baseline rest condition. The substantial differences between playing chess and doing nothing mean that a sprawling network of active brain areas is evident here.
Let's be more specific and try to isolate some parts of the brain that are more uniquely involved in chess playing rather than broader cognitive and perceptual abilities. The researchers did that by using a different contrast that matches more low-level task demands across conditions. In the figure below, you can see the results of comparing just the Checkmate and Rule Retrieval conditions to the Visual Search task. Unlike the Resting contrast up above, this new contrast matches a bunch of simpler processes across both halves of the comparison: In both cases, you're seeing a chessboard. In both cases, you are probably naming and recognizing the pieces to perform accurately. In both cases, you have to come up with some motor response to meet the task demands. Because those processes are being carried out in both parts of the contrast, we wouldn't expect to see significant differences in activity in brain areas that support those tasks. What's left? Hopefully the brain areas that are specifically active when you're working out potential moves, including the possibility of a forced mate. If you take a look at the figure below, you can see that it's a lot less busy than the first one: Instead of that whole jumble of active regions, this is much pared down - if you consider just the regions that are still significant when implementing something called family-wise error correction (a technical step that is important but beyond our scope, I think), it's really just one spot! One spot up there in the left parietal lobe. One spot potentially close to the place where AB's surgeons need to resect a tumor. Now what?
Figure 7 (adapted from Cepero-Escribano et al., 2024) - A finer-grained comparison of chess-playing ability as assessed via move and checkmate evaluations compared to a visual search task on a chessboard. This contrast reveals significant activity in the left parietal cortex rather than a more distributed network of brain areas.
Bespoke brain surgery via Electrical Stimulation Mapping
One thing about fMRI that's important to keep in mind is that while it is very good at telling you what parts of the brain are more active in one condition vs. another, it still has a limited spatial resolution. We talk about fMRI data in terms of voxels, which are the volumetric equivalent of the pixels that you're probably more familiar with on your phone, laptop, or tablet display. When I was first learning to do fMRI experiments, a state-of-the-art voxel size was a cube that was about 3mm to a side. These days, something more like 1mm to a side is much more common, but that's still not necessarily a fine enough level of detail to be as careful as we'd like to be for Patient AB. Enter a classic technique from foundational 20th-century neurological research - electrical stimulation mapping or ESM.
The gist of ESM is that because neural activity is electrical in nature, you can attempt to understand what the neurons in a particular part of the cortex do by manipulating the electrical signals in that area yourself to see what happens. Specifically, one can place an electrode in part of the brain that you're interested in and inject current there to see either what the patient experiences when you do this, or to see what activities you disrupt by altering the electrical activity there. I don't think I have the space for a full history lesson or anything, but suffice it to say that some of the earliest studies using this technique helped establish the layout of motor and somatosensory cortex by eliciting specific movements (e.g. isolated limb motions) or localized sensations (a feeling of pressure on part of the arm, e.g.) as the stimulation site was moved across the cortical surface. Critically, it's possible to carry out this procedure on an awake patient who is about to undergo brain surgery - after exposing the part of the brain you need to work with, you can begin stimulating and testing the patient behaviorally to understand where eloquent cortex is and isn't relative to different tasks. This is exactly what AB's surgeons did before resecting his tumor - what nearby parts of his left parietal lobe contributed to his bilingual language abilities? What about his programming skill? And of course, for our purposes, what about his chess playing? I've spared you the rather gory version of this figure in case some of you are a bit squeamish, but a schematic view of what they found is presented below.
Figure 8- (adapted from Cepero-Escribano et al., 2024) The results of ESM applied to Patient AB. Eloquent cortex supporting each of these abilities was found in the labeled regions near the surgical aperture, indicating how to try and preserve those abilities while still targeting his tumor.
Right there, labeled in yellow as site #7 - there is chess ability in Patient AB's brain. Stimulating there while he tries to carry out those behavioral tasks leads him to have serious difficulty, though stimulating at spots just adjacent has no such effect. That level of detail meant that his surgeons were able to define a surgical aperture around these various portions of eloquent cortex, remove the tumor, and (hopefully) preserve the skills that AB had developed over decades of experience and training. I say "hopefully" there, but at least with regard to the chess playing - it worked! Patient AB reported months after the surgery that he had been able to maintain his 1950 ELO rating after subsequent OTB play.
I started this post by taking some pains to tell you why I think you have to be careful about your reasons for wanting to study brains if you want to understand the mind. What I love about this case study is that I feel like it includes all the things that I think are important about understanding the brain itself. Finding locations in the left parietal lobe that support AB's chess playing aren't just random factoids to be logged in a modern phrenological chart - instead, they provide important insights into what chess playing is. We know a good bit about the kinds of spatial cognition that appear to happen in that part of the brain, so seeing this region identified in AB (and seeing its proximity to things like his programming ability) helps us make brain-based connections between cognitive functions that help reveal the nature of chess cognition. This kind of insight, which I talked about some in myspatial cognition post, can serve as the starting point for more and more specific hypotheses about chess expertise as a unique process and a process that likely recruits a suite of more general cognitive abilities. That, to me, is the promise of cognitive neuroscience research. Besides this, I find the human element of this case study quite affecting: Here's a guy, about my age, who has had something life-changing happen to him and he's hoping there's a way to keep it from changing his mind too drastically. By looking carefully for where chess-playing was in his brain (not just brains in general!) this group was able to help make that a reality.
Support Science of Chess posts!
Thanks for reading! If you're enjoying these Science of Chess posts and would like to send a small donation ($1-$5) my way, you can visit my Ko-fi page here: https://ko-fi.com/bjbalas - Never expected, but always appreciated!
References
Weisberg DS, Keil FC, Goodstein J, Rawson E, Gray JR. The seductive allure of neuroscience explanations. J Cogn Neurosci. 2008 Mar;20(3):470-7. doi: 10.1162/jocn.2008.20040. PMID: 18004955; PMCID: PMC2778755.
Cepero-Escribano, V., Cerda-Company, X., León-Cabrera, P., Olivé, G., Cucurell, D., Gasa-Roqué, A., Gabarrós, A., Naval-Baudin, P., Camins, À., Rico, I., Fernández-Coello, A., Sierpowska, J., & Rodríguez-Fornells, A. (2024). Can the knight capture the queen? The role of supramarginal gyrus in chess rule-retrieval as evidenced by a novel combined awake brain mapping and fMRI protocol. Cortex; a journal devoted to the study of the nervous system and behavior, 178, 235–244. Advance online publication. https://doi.org/10.1016/j.cortex.2024.05.022
View more blog posts by NDpatzer
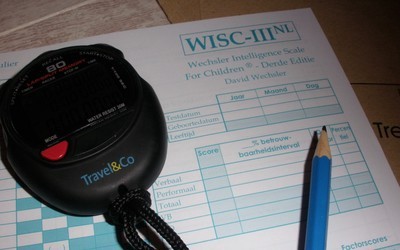
Science of Chess: A g-factor for chess? A psychometric scale for playing ability
How do you measure chess skill? It depends on what you want to know.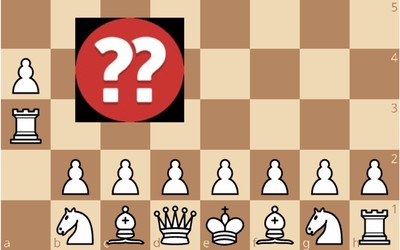
Science of Chess (kinda?): Viih_Sou's 2. Ra3 and a modest research proposal
Take the 2. Ra3 Challenge! For Science!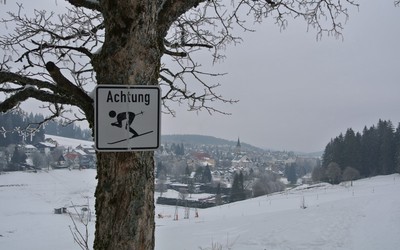
Science of Chess - Achtung! Einstellung!
Pattern recognition is great, but the Einstellung effect can turn a master into a patzer (sort of).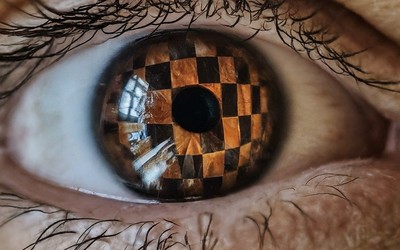